
ORIGINAL ARTICLE
Individual variability in response to a single sickling event for normal, sickle cell, and sickle trait erythrocytes
MICHAEL TARASEV, MARINA MUCHNIK, LUCIA LIGHT, KENNETH ALFANO, and SUMITA CHAKRABORTY
ANN ARBOR, MICH AND TORONTO, ONTARIO, CANADA
Hemoglobin S (Hb-S) polymerization is the primary event in sickle cell disease causing irreversible damage to red blood cell (RBC) membranes over repeated polymerization cycles. A single polymerization triggered by a hypoxic environment was reported to result in reversibly (upon reoxygenation) decreased RBC deform- ability and increased mechanical fragility (MF). Individualized responses have not been reported, although RBC fragility can vary significantly even among healthy in- dividuals. This study evaluates individual variability in response to a single hypoxia- induced sickling event, through changes in RBC MF. Blood was drawn from 10 normal (AA), 11 sickle cell (SS), and 7 sickle trait (AS) subjects—with Hb-S fraction, osmotic fragility, and medical history also collected. Mechanical stress was applied using a bead mill at 50-Hz oscillation for 0.5–30 minutes. MF profiles here give percent hemo- lysis upon successive durations of stressing. MF was measured for AA, SS, and AS cells—each equilibrated (1) with air, (2) with nitrogen in an anaerobic chamber, and (3) with air after the hypoxic event. While AA subjects exhibited significantly different changes in fragility upon hypoxia, in all cases there was recovery to close to the initial MF values on reoxygenation. For AS subjects, recovery at reoxygenation was observed only in about half of the cases. Fragility of SS cells increased in hypoxia and decreased with reoxygenation, with significantly variable magnitude of recov- ery. The variability of response for individual AS and SS subjects indicates that some are potentially at higher risk of irreversible hypoxia-induced membrane damage. (Translational Research 2016;-:1–12)
Abbreviations: RBC ¼ Red blood cells; SCD ¼ Sickle cell disease; SCA ¼ Sickle cell anemia; SCT ¼ Sickle cell trait; HU ¼ hydroxyurea; Hb ¼ hemoglobin; AA ¼ normal cell, ie, hemoglobin with beta chain gene with 2 normal alleles; AS ¼ sickle trait cell, ie, hemoglobin with beta chain gene with 1 normal and 1 abnormal alleles; SS ¼ sickle cell, ie, hemoglobin with beta chain gene with 2 abnormal alleles; AUC ¼ Area under the curve; OXY ¼ open to air conditions; HYPOXY ¼ hyp- oxic conditions; Oxy-Hb ¼ oxygenated hemoglobin; Deoxy-Hb ¼ deoxygenated hemoglobin; Met-Hb ¼ oxidized hemoglobin; Hb-F ¼ fetal Hb; MF ¼ Mechanical fragilit
From the Blaze Medical Devices, Ann Arbor, Mich; Ontario HIV Treatment Network, Toronto, Ontario, Canada.
Submitted for publication March 31, 2016; revision submitted September 15, 2016; accepted for publication September 17, 2016.
Reprint requests: Michael Tarasev, Blaze Medical Devices, 330 E. Liberty Street, Lower Level, Ann Arbor, MI 48104; e-mail:
1931-5244/$ - see front matter © 2016 Elsevier Inc. All rights reserved. http://dx.doi.org/10.1016/j.trsl.2016.09.005

AT A GLANCE COMMENTARY
Tarasev M, et al.
Background
Severity of sickle cell disease is highly heteroge- neous, with severity possibly modulated by sickle RBC membrane alterations. Little data is available on patient-specific changes. Mechanical fragility can be used to probe membrane resistance to me- chanical stress. Translational Significance The ability of RBC to recover after a single hypox- ic event varies significantly among sickle cell ane- mia subjects (as demonstrated by changes in cells’ mechanical fragility profiles), potentially indica- tive of differences in complication risks and condi- tion severity. If clinically validated, this approach could offer a practical test indicative of which pa- tients are more at risk for extensive pathology, thus allowing better-targeted and potentially timelier interventions.
INTRODUCTION
Sickle cell disease (SCD) is one of the most common hereditary blood disorders, affecting up to 100,000 peo- ple in the U.S. alone, mostly African-Americans, with up to 2 million people carrying the sickle cell trait (SCT).1 Symptoms include chronic anemia, pain crises, pulmonary hypertension and acute chest syndrome, stroke, splenic and renal dysfunction, and susceptibility to bacterial infections. Treatment focuses on alleviating symptoms and preventing complications, with blood transfusions often used in severe anemia cases.
Hemoglobin S (Hb-S) polymerization is the primary event in SCD, resulting, over repeated cycles of poly- merization and morphological changes, in irreversible damage to red blood cell (RBC) membranes—develop- ment of sickle- or crescent-shaped cells that tend to block blood vessels.2 Sickle cell anemia (SCA) patients are characterized by 2 abnormal alleles of the hemoglo- bin (Hb) beta gene (SS Hb phenotype), while SCT indi- viduals have 1 normal allele (A) and 1 abnormal (S) allele of the Hb beta gene (AS Hb phenotype).
SS RBC exhibit an array of defects and alterations of the RBC membrane which profoundly affect cell biochemical and biomechanical functions.3 Even when not sickled, an oxygenated SS cell is both less deform- able and less able to withstand mechanical stress (eg, Barodka et al4 and MacCallum et al5). Unlike SS-type
cells from SCA patients, AS-type cells seem to be similar to those from normal subjects (AA Hb phenotype cells).6 However, Hb-S polymerization, promoted by low oxy- gen tension and anticoagulant in collected blood, could result in impaired filterability and deformability of cells from SCT individuals as well,4,7 although an absence of the effect on membrane properties also had been reported (eg, Lawrie et al8). These observations should be considered with the understanding that RBC deform- ability and fragility reflect nonidentical membrane prop- erties, with mechanical fragility (MF) reflecting cell stability under mechanical shear stress. Interestingly, Chasis and Mohandas9 reported that deformability and stability of RBC membranes are in fact regulated through different interactions of skeletal proteins. For deform- ability at least, it was demonstrated that cellular dehydra- tion and resultant increase in Hb intracellular concentration, rather than altered membrane properties, are the primary determinants of decreased deformability and the abnormal rheological behavior of sickled RBC.10 Even in the course of normal physiological func- tioning, low oxygen tension can lead to Hb-S polymer- ization with a resultant loss of RBC deformability.11 It should be noted that loss of deformability may occur at higher pO2s than cell morphological sickling, with sensitivity to oxygen being different between cell sub- populations based on density fractioning.12 Deeper pO2 decline induces reversible or irreversible sickling, possibly depending on the reversibility of the Hb-S binding to the cdb3 complex, with corresponding in- crease in cell membrane rigidity.13,14
Sickle cell membrane properties, including deformabil- ity and rigidity, play an important role in both adhesive and obstructive events, contributing to the pathophysiology of SCA,15,16 with sickling shown to correlate with the severity of major SCA complications.17 In addition to vaso-occlusion, destabilization of the RBC membrane and cell sickling result in premature RBC destruction and release of Hb which was suggested to contribute to the pathology by limiting nitric oxide bioavailability18 and promoting oxidative damage.19 Hemolysis (Hem), likely dependent on cell membrane properties including its rigidity and fragility, was suggested as the possible proximate cause of SCA complications such as pulmonary hypertension, priapism, ulcers, and stroke20 and was further linked to mortality.21
While repeated sickling is shown to result in irrevers- ible membrane deformation, only recently changes due to a single sickling event were investigated. Presley et al22 reported that in both SS and AS cells, a single polymerization triggered by a hypoxic environment re- sulted in reduced deformability and elevated fragility of RBC. In AA cells, hypoxia was not observed to induce any change. However, extremely high standard

deviations (SDs) of RBC MF, especially for deoxygen- ated samples (coefficients of variability up to 85%, on n 5 3), imply that there were significant differences among samples from individual subjects. Reoxygenation showed no effect on AA cells, but resulted in return of MF properties in SS and AS cells to prehypoxia values. Variability, however, remained high, with coefficient of variation in the 39%–83% range. RBC mechanical prop- erties have also been reported to differ among both normal23 and SS individuals,24 yet there is little data on patient-specific differences in RBC response (eg, magni- tude and reversibility) to insult and/or treatment. This study evaluates individual variability in response to a sin- gle hypoxia-induced sickling event through changes in RBC MF, as an early step in exploring a hypothesis that person-to-person variability in individuals’ RBC MF, and its changes due to hypoxia which could be potentially significant for medical decision-making in re- gards to individuals with SCA and/or those carrying SCT
MATERIALS AND METHODS
Study design. Blood samples (AA, AS, and SS) were obtained from volunteers using 3.2% sodium citrate tubes during routine clinic visits by SS donors reporting no vaso-occlusive–type symptoms. Research was car- ried out according to The Code of Ethics of the World Medical Association (Declaration of Helsinki). Blood donations were collected from consented subjects, in accordance with the ethical standards of the responsible committee on human experimentation (IRB, BloodCen- ter of Wisconsin). After collection, samples were stored at 40C and shipped on ice to the testing laboratory by overnight express shipping, with testing commencing in 22 6 4 hours. Independent experiments indicated no significant changes in auto-Hem and MF within the first 48 hours after collection (unpublished observations). Blood samples were centrifuged for
5 minutes at 1300 rcf on an Eppendorf 5417C centrifuge; the supernatant was removed and replaced with an equal volume of AS3 at pH 5.75. Samples were further diluted to a total Hb concentration (HbT) of 1.7 g/dL (corresponding to about 4% hematocrit) with the same solutions as above. Diluted sample was gently agitated and aliquoted into 2-mL low-retention centrifuge tubes at 330 mL per tube. The study design is graphically represented in Fig 1. Each blood sample was tested as follows: (1) oxygen- ated (OXY)—exposed to air; (2) deoxygenated (HY- POXY)—equilibrated with nitrogen and thus in essentially oxygen-free (hypoxic) conditions, with oxygenated form of Hb (Oxy-Hb) converted to deoxy- genated form (Deoxy-Hb); and (3) reoxygenated (RE- OXY)—equilibrated with air after being subjected to
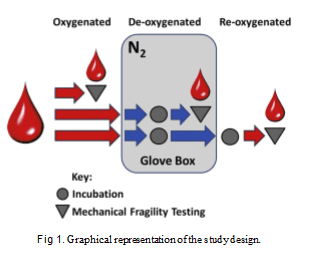
1 round of hypoxia, with Deoxy-Hb fully converted back to Oxy-Hb form through equilibration with air. To maintain deoxygenated conditions, the samples, diluted and aliquoted as described above, were incu- bated for 2 hours with gentle mixing in a glove box made essentially oxygen-free by repeated (33) purging with nitrogen. MF testing (see below) on these samples was performed in the glove box, after fractional Oxy-Hb concentration was below 10%, as confirmed spectro- photometrically using an Ocean Optics STS spectro- photometer (Dunedin, Fla). Reoxygenation control sample was subjected to the same deoxygenation pro- cess and then reoxygenated with room air by gentle mixing for 30 minutes. Reoxygenation to essentially 100% Oxy-Hb was also confirmed using the STS spec- trophotometer.
Data collected. Subject demographics including age and sex, as well as relevant medical history including hydroxyurea (HU) treatments and transfusion history, were collected. All samples were analyzed for relative concentrations of the main Hb types (Hb types A1, A2, S, C, and F–with analyses performed by Dynacare Laboratories, Wis.). In addition, RBC osmotic fragility was determined by the BloodCenter of Wisconsin labo- ratory, Wis. and recorded as a profile representing induced Hem as a function of sodium chloride concen- tration (P50).
Mechanical fragility test. Mechanical stress was applied to RBC samples with the use of a TissueLyser LT (Qiagen, Valencia, Calif) bead mill at an oscillation frequency of 50 Hz in the presence of 1 7-mm diameter stainless steel ball for predetermined durations. Subsamples were subjected to such stress at 12 different durations (ranging from 0 sec to 30 min) to ensure a wide range of achieved Hem levels. The sample holder of TissueLyser was modified to allow air cooling while in operation, which resulted in sample

temperature stabilization to within 20 of the starting temperature. Unlysed cells were pelleted by centrifuging the samples for 5 minutes at 1300 rcf on an Eppendorf 5417C centrifuge. Samples of the supernatant were collected and used for spectral analysis. Hemolysis assessment. Hem was determined based on the difference in absorbance at 576 nm, a wavelength of oxygenated Hb maximum, and absorbance at 685 nm. It was expressed as a fraction of free Hb (HbF) relative to HbT according to Formula 1 which includes the correction for sample hematocrit as detailed by Sowemino-Coker.25
HbT for each diluted RBC sample was determined by subjecting a small (350 mL) aliquot to a short (40s) ul- trasound treatment (0.15 W) on ice. In control experi- ments, such treatment was shown to fully hemolyze RBC. For determining auto-Hem (Hem prior to the application of mechanical stress), small (20 mL) sam- ples of undiluted sample were centrifuged for 5 min at 1300 rcf, supernatants were collected, and the Hb con- tent was measured spectrophotometrically. Spectro- scopic measurements were performed with a NanoDrop N1000 spectrophotometer (ThermoFisher, Wilmington, Del).
Relative contributions of different Hb forms— including oxygenated (Oxy-), deoxygenated (Deoxy-), and oxidized (Met-) Hbs—were determined when necessary by fitting base-line adjusted sample absor- bance spectra with a linear combination of molar extinc- tion coefficients for Oxy-, Deoxy, and Met-Hb forms in the near-UV-Vis (450–685 nm) range. Awavelength at a local absorbance minimum of Oxy-Hb, 685 nm, was used as a baseline. The fitting was performed using a Solver function in Excel (Microsoft, Redmond, Wash). For Oxy-Hb and Deoxy-Hb forms, extinction coefficients reported by Prahl26 were used. Extinction of oxidized Hb (Met-Hb) was determined in this labora- tory, with reference to values reported previously by van Assendelft and Zijlstra.27,28 For samples containing noticeable amounts of Met-Hb, absorbance observed at 576 nm HF was adjusted based on the difference in extinction coefficients of Oxy-Hb and Met-Hb at 576 nm and 630 nm, the wavelengths of absorbance maxima of Oxy-Hb and Met-Hb, to represent combined Hb absorbance.
RBC fragility profiles are defined here as the cumula- tive incremental induced Hem (ie, beyond auto-Hem) resulting from applied stress of varying durations. Un- like single-point measurements that use a single stress duration at a single stress intensity, as implemented
Osmotic fragility was evaluated based on P50, which induces 50% Hem in the sample, and S50, the slope of the change in Hem at the P50 point. The values were calculated based on the best-fit low polynomial.
Statistical analysis. Data are presented in the form of of 0.05 with Bonferroni correction for multiple
comparisons were used to test for significance where appropriate. Unless specified otherwise, P-values in the text are those after the Bonferroni correction. The study design required testing RBC samples of the same subjects under 3 different conditions (OXY, HYPOXY, and REOXY). For all subjects (AA, AS, and SS), the results of the tests performed in those conditions were highly correlated, with statistically significant correlation coefficients ranging from 0.7 to 0.9 for AA, 0.9 between HYPOXY and REOXY for AS, and 0.7 between OXY and REOXY for SS. (Other correlation coefficients are either of marginal significance or not significant.) This correlation must be accounted for in statistical analysis. Consequently, the repeated measures ANOVA model using mixed model approach, similar to that described previously,17 was used for determining statistical significance between the conditions. Such an approach is better suited for the present case than ordinary linear regression or ordinary 1-way ANOVA, as ordinary analysis would not account for correlation between repeated measurements from the same subject. However, when a single condition was analyzed, ordinary linear regression was used. The analysis was performed using SAS 9.4 (SAS Institute, Cary, N.C.).24
Materials. Albumin was from RPI (Mount Pleasant, Ill); all other materials were from Sigma (St. Louis, Mo.).
RESULTS
Recruited subject overview. 30 subjects were recruited for the study. Of those, 10 were normal subjects with no known pathologies (AA), 7 had sickle trait (AS), and 13 had SCD. Of the 13 SCD subjects, 2 were excluded from the study as Hb analysis revealed SC-type Hb. One of the remaining SS samples exhibited clots on arrival, leaving 10 SCA or SS samples for analysis. For AA subjects, Hb concentration was found to be the highest, closely followed by that from AS subjects,

Table I. Clinical characterization of participants, mean (SD)
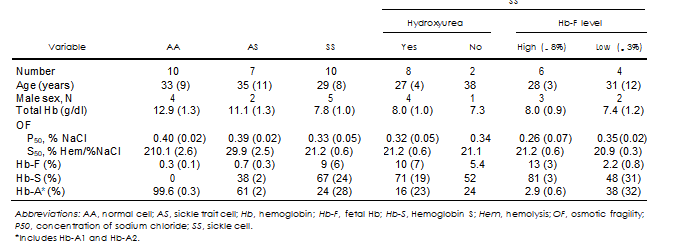
and markedly lower for SS subjects (Table I). These differences were statistically significant (P , 0.05). As anticipated, very low fetal-type (F) and no sickle- type (S) Hb was observed for AA subjects, with samples from AS subjects exhibiting significant (about 38%) content of Hb-S. Concentrations of both F- and S- types of Hb varied significantly for SS subjects, with the breakdown for patients who were receiving (8 out of 10) and not receiving (2 of 10) HU shown in Table I. Efficacy of HU treatment, as evaluated based on an increase in fetal Hb (Hb-F), varied significantly among patients, with no clear relationship observed between Hb-F fraction and HU dose (between 0 and 2000 mg/d), subject’s gender, age, or Hb content at collection; however, compliance with the treatment had not been assessed. Notably, elevated Hb-F was detected in all SS samples even those not currently receiving HU. No correlation between Hb types and gender or age was observed.
Normal subjects. While on average, the AUC value for AA subjects was 17.2 6 4.5, these individuals appeared to
fall into 2 distinct groups: those exhibiting low (AUC of 12.1 6 0.8) and high (AUC of 20.7 6 1.1) RBC MF levels (Table II and Fig 2). The difference between the 2 groups was statistically significant (P much less than 0.05) for all testing conditions (OXY, HYPOXY, and REOXY). For AA subjects, no correlation was observed between the high/low RBC MF at any of the 3 conditions and age, gender, or initial Hb concentration. However, osmotic fragility, as represented by P50, but not S50, was significantly correlated with RBC MF for OXY (P , 0.04, R2 5 0.43) and REOXY (P , 0.04, R2 5 0.45) samples. No such correlation was detected for the HYPOXY condition.
For all AA subjects, hypoxia resulted in elevated RBC MF, with reoxygenation substantially reversing this increased susceptibility to Hem. However, the magni- tudes of these effects varied between the 2 groups (ie, between those with initially ‘‘high’’ and ‘‘low’’ RBC MF). The low-fragility group exhibited a pronounced increase in RBC MF on hypoxia; however, while reduced, RBC MF stayed elevated upon reoxygenation
Table II. Averaged values for mechanical fragility of RBC
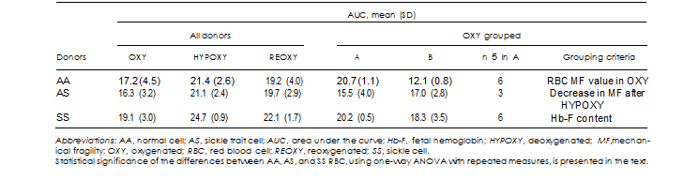

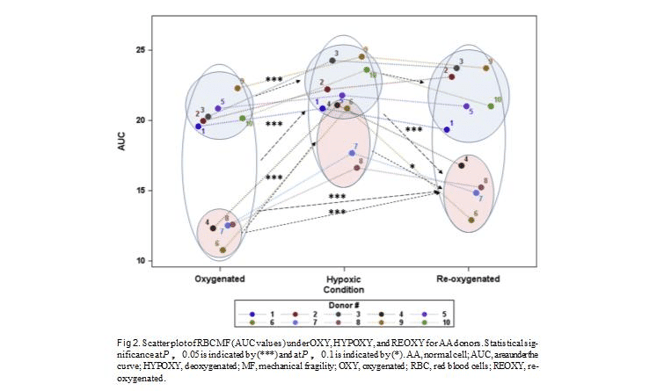
as compared to OXY. Statistical analysis shows that while the differences between OXY and HYPOXY are statistically significant (P , 0.05), those between HY- POXY and REOXY are not (P 5 0.2), with the compos- ite result that differences between OXYand REOXYare only of marginal significance (P 5 0.05).
While for some subjects in the high-fragility group (eg, Subject #5, Fig 2), hypoxia and reoxygenation re- sulted in essentially no net changes in RBC MF; on average in this group, there is still a difference between OXY and HYPOXY (P 5 0.014). However, the differ- ences between HYPOXYand REOXY, and more impor- tantly between OXY and REOXY, are not significant (P 5 0.112 and P 5 0.123, respectively).
Sickle trait subjects. Initial (OXY) RBC MF of AS sub- jects was not significantly different from that of the average of all AA subjects (Table II); however, at the listed SD and sample sizes, the power of analysis is not adequate for definitive conclusions. Nevertheless, when considered separately in comparison with each of the 2 groups of AA subjects, MF did show statistical significance: AS fragility was higher when compared to AA for the low-MF group (P 5 0.01) and lower when compared to AA for the high-MF group (P 5 0.01). At the low power of the comparison, osmotic fragility of AS subjects was not found to be different from that of
SS subjects (Table I). It can be noted that OXY RBC MF of AS subjects exhibited about equal numbers of ‘‘high’’ and ‘‘low’’ values (Fig 3) which can be viewed as parallel to that observed in AA subjects; the small sample size, however, precludes statistical analysis of this observation.
Hypoxia resulted in a statistically significant increase in RBC MF (P 5 0.004), while reoxygenation resulted in only a marginal MF decrease (P 5 0.1), with the result that RBC MF at REOXY remained on average signifi- cantly different from that at OXY (P 5 0.029); the latter 2 results have significantly lower power which makes those results not very reliable. Notably, use of a less- conservative Tukey-Kramer correction resulted in adjusted P-values (much greater than 0.05) for all the 3 transitions, thus confirming that the AS group sample size may have been insufficient to properly test for these changes.
Based on the magnitude of recovery of RBC MF after hypoxia, AS subjects can be conditionally separated into 2 groups, representing ‘‘high’’ (40%–70%) and ‘‘low’’ (under 30%) fractions of cell, based on their ‘‘re- covery’’ (regained stability) after hypoxia. No correla- tion between those and the magnitude of the RBC MF at OXY condition, gender, age, osmotic fragility param- eters, total Hb concentration, or Hb-S fraction was observed.

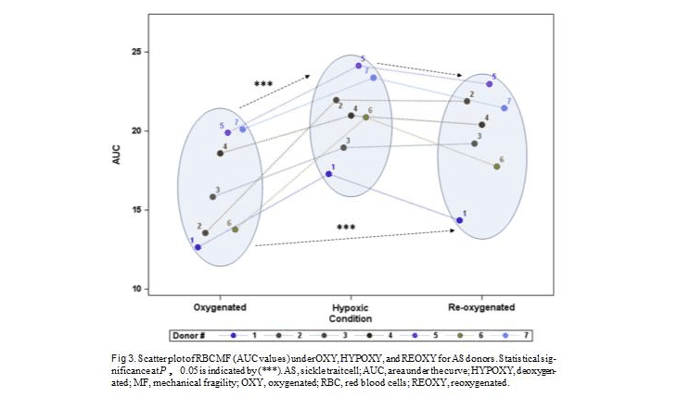
as compared to OXY. Statistical analysis shows that while the differences between OXY and HYPOXY are statistically significant (P , 0.05), those between HY- POXY and REOXY are not (P 5 0.2), with the compos- ite result that differences between OXYand REOXYare only of marginal significance (P 5 0.05).
While for some subjects in the high-fragility group (eg, Subject #5, Fig 2), hypoxia and reoxygenation re- sulted in essentially no net changes in RBC MF; on average in this group, there is still a difference between OXY and HYPOXY (P 5 0.014). However, the differ- ences between HYPOXYand REOXY, and more impor- tantly between OXY and REOXY, are not significant (P 5 0.112 and P 5 0.123, respectively).
Sickle trait subjects. Initial (OXY) RBC MF of AS sub- jects was not significantly different from that of the average of all AA subjects (Table II); however, at the listed SD and sample sizes, the power of analysis is not adequate for definitive conclusions. Nevertheless, when considered separately in comparison with each of the 2 groups of AA subjects, MF did show statistical significance: AS fragility was higher when compared to AA for the low-MF group (P 5 0.01) and lower when compared to AA for the high-MF group (P 5 0.01). At the low power of the comparison, osmotic fragility of AS subjects was not found to be different from that of
SS subjects (Table I). It can be noted that OXY RBC MF of AS subjects exhibited about equal numbers of ‘‘high’’ and ‘‘low’’ values (Fig 3) which can be viewed as parallel to that observed in AA subjects; the small sample size, however, precludes statistical analysis of this observation.
Hypoxia resulted in a statistically significant increase in RBC MF (P 5 0.004), while reoxygenation resulted in only a marginal MF decrease (P 5 0.1), with the result that RBC MF at REOXY remained on average signifi- cantly different from that at OXY (P 5 0.029); the latter 2 results have significantly lower power which makes those results not very reliable. Notably, use of a less- conservative Tukey-Kramer correction resulted in adjusted P-values (much greater than 0.05) for all the 3 transitions, thus confirming that the AS group sample size may have been insufficient to properly test for these changes.
Based on the magnitude of recovery of RBC MF after hypoxia, AS subjects can be conditionally separated into 2 groups, representing ‘‘high’’ (40%–70%) and ‘‘low’’ (under 30%) fractions of cell, based on their ‘‘re- covery’’ (regained stability) after hypoxia. No correla- tion between those and the magnitude of the RBC MF at OXY condition, gender, age, osmotic fragility param- eters, total Hb concentration, or Hb-S fraction was observed.

Sickle cell subjects. If compared with using a whole da- taset, initial (OXY) RBC MF of sickle cell subjects was not significantly different (P 5 0.3) from that of the average of all normal subjects (Table II). However, when comparing separately to both high and low RBC MF normal subjects, analysis reveals that while MF of SS subjects was still not significantly different from MF of high-fragility AA donors (P 5 0.1), it was significantly (P , 0.0001) different from that of low- fragility AA donors. Osmotic fragility of SS subjects was significantly different, in both the P50 and S50 values, from that of AA and AS subjects (Table I). While the P50 value exhibited a relatively modest change (within 2 SD), the change to the slope of the osmotic fragility profile was much more pronounced (up to 10 fold). It should be noted that most SS samples exhibited 2 phases in osmotically induced RBC Hem, with typically a much smaller and slower phase at lower salt concentrations, followed by a larger and faster phase.
Hypoxia resulted in a statistically significant increase in RBC MF (P much less than 0.01) when assessing the whole SS subject dataset, with the follow-up reoxygena- tion inducing a statistically significant MF decrease (P 5 0.005). Resultant RBC MF at REOXY was on average significantly different from that at OXY (P 5 0.02) (see Fig 4 and Table II). However, while SS subject response to hypoxic event resulted in nearly
uniform increase in RBC MF, post-event recovery was markedly varied. While for some individuals, for example subject 4 (Fig 4), reoxygenation brought about a decrease in MF down to prehypoxic level; for other in- dividuals, for example, subject 6 (Fig 4), who had RBC MF similar to subject 4 at OXY and HYPOXY condi- tions, reoxygenation did not result in recovery (indi- cating irreversible changes to RBC membrane stability). No statistically significant correlations were observed between RBC MF at any of the conditions and subject gender, age, hemoglobin content, osmotic fragility pa- rameters (P50 and S50), HU dose, or Hb-F content (except for a trend toward significance at P 5 0.07 when corre- lated with the REOXY condition). Similarly, those did not exhibit a correlation with an increase in MF (decrease in cell ability to withstand mechanical stress) brought about by hypoxia. Age, Hb content, and osmotic fragility parameters likewise were not significantly correlated with changes in MF upon post-hypoxic RBC reoxygena- tion. However, gender was found to be an independent predictor (P 5 0.05, R2 5 0.4) of how closely RBC MF returns to its initial, prehypoxia level after the hypox- ic event (the difference between RBC MF at OXY and REOXY), with males exhibiting better and less- variable recovery to the original values.
In addition, Hb-F content was also found to be a pre- dictor of post-hypoxia recovery. A strong correlation

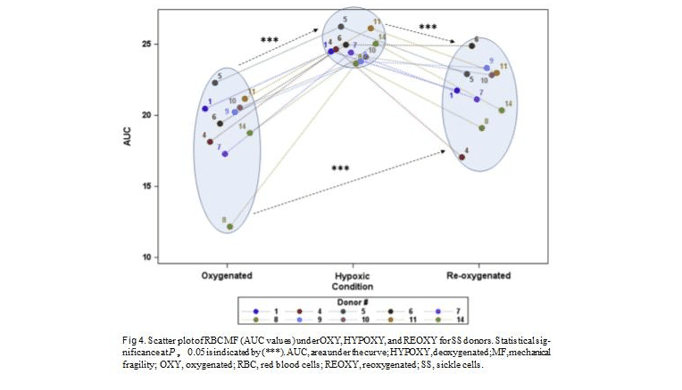
(P , 0.01, R2 5 0.66) was observed between its levels and the magnitude of change in RBC MF upon sample reoxygenation. However, as Hb-F was not a predictor for the initial OXY RBC MF values, it was poorly corre- lated with the OXY-REOXY difference, reflecting how closely cells’ MF reverts to prehypoxia values. Correla- tion to Hb-F trended toward significance (P 5 0.056) when the magnitude of recovery was normalized by the magnitude of hypoxia-induced fragility increase [(DEOXY 2 REOXY)/(DEOXY 2 OXY)].
Overall, SS subjects in this study could be subdivided into 2 groups: those with ‘‘low’’ (,3%), and those with ‘‘high’’ (.8%) Hb-F content. No relationship was observed with subject age, gender, initial Hb concentra- tion, or even HU dosage, but given the small sample sizes for the subgroup analysis, the power is not suffi- cient for statistical inference. A difference between initial (at OXY) RBC MF values for the 2 groups was also not observed.
DISCUSSION
It had been demonstrated previously that reversible binding of Band 3 anion exchange protein (cdb3) and Deoxy-Hb, but not Oxy-Hb, results in transient rupture of the cdb3-ankryn bridge and increased cdb3 diffusion,
thus providing an oxygen tension–dependent switch to membrane properties.31 It had also been suggested that disruption of junctions in ankyrin-cdb3 and spectrin-actin-protein 4.1 complexes could lead to membrane fragmentation under sufficiently high shear stress.9 Conversely, less-tight association of ankyrin with the cell membrane, and thus disassociation be- tween the phospholipid bilayer and the underlying spectrin-actin cytoskeleton, can be expected to weaken the RBC membrane on cell deoxygenation, making it potentially less elastic and at the same time potentially less resistant to mechanical stress.
As Hb-induced structural membrane rearrangements appear to be a part of the normal physiological function, reoxygenation of the cell should trigger the reverse effect: breakage of the bond between cdb3 and hemoglobin, now in oxy-Hb form, and reattachment of ankyrin, with the RBC membrane potentially better able to withstand me- chanical stress. Indeed, while the transition to hypoxic conditions in the present study was associated with higher propensity to stress-induced Hem (higher RBC MF), re- oxygenation brought about cell recovery with a decline of RBC MF toward initial (prehypoxic insult) values.
Related to this result, RBC membrane properties were reported to remain essentially unchanged at physiolog- ical hypoxia levels,14 with their fragility apparently

unaffected even by severe hypoxia and follow-up cell reoxygenation.22 (However, in the latter case, as mentioned, the data are presented as averages and are based on very small (n 5 3) sample size with very high SD, raising the question of potential variability among different individuals, and the need for pairwise comparison in the analysis.) In additional, while a num- ber of authors reported that for AA individuals hypoxia does not cause significant changes in RBC deformabil- ity, others report that with normal RBC, deformability increases at higher oxygen tension and declines signifi- cantly at hypoxic conditions (eg, Hakim and Macek32 and Uyuklu et al33). Further studies would be required to understand how these contradictory experimental re- sults correlate with the mechanistic considerations out- lined above.
It is well known that a variety of genetic factors, including G6PD variants, can influence RBC properties (eg, Maha34 and Soranzo35). Cells also can be markedly influenced by lifestyle, and in particular, diet and exer- cise have been identified as factors significantly affecting the ability of cells’ membranes to withstand stress and to deform.36-38 (Incidentally, in patients with SCD, these factors can significantly affect the severity of the disease39).
Interestingly, in this study, we observed 2 groups of AA individuals that differed in both the initial MF of their RBC as well as in the cell reaction to hypoxia. The ‘‘low’’ initial (OXY) MF group exhibited a marked increase in MF on hypoxia, with cells only partially recovering upon reoxygenation. In contrast, the RBC with initially high MF showed only a moderate increase upon deoxygenation, and seemed to recover fully when reexposed to air. It is conceivable that the difference in response to hypoxia between these 2 groups may have been substantially due to differences in levels of phys- ical fitness. (While study of any such correlation was outside the scope of the present work, this hypothesis may merit further investigation.)
When Hb-S is present in RBC, the often- interconnected effects of oxidative damage accumulating during repeated sickling, Hb-S interaction with mem- brane proteins, and cell dehydration/shrinkage, can cause marked alterations of biomechanical membranes. In particular, SS RBC are attributed with weakened interac- tion between the membrane lipid bilayer and its skeletal proteins, likely due to reduced spectrin-Ankyrin bind- ing.40 Destabilization of the membrane bilayer and mi- crovesicle shedding, which were shown to be greatly elevated in sickle cells even with no crisis, could also affect cell membrane stability.41,42
Although SCT condition is typically considered asymptomatic, sometimes it can be associated with complications similar to those in SCA, including,
among others, chronic kidney disease, hematuria, splenic infarction, and venous thromboembolic events.43-45 SCT complications often relate to exertion and a resultant low-oxygen/hypoxic cell environment, and while there is typically no Hb polymerization in AS cells under physiological conditions, such can still occur in hypoxic tissues, and had been shown to be inducible by hypoxia in vitro.46 It is not well understood why some people with SCT may be more susceptible to those complications than are others. In view of the ob- servations here, it is conceivable that susceptibility to complications could be linked to heightened propensity of the RBC membrane for irreversible changes under hypoxia. The question of possible changes in AS cell membrane properties has additional relevance for blood banking and transfusion medicine. While some authors report that blood storage and post-transfusion in vivo cell survival are not significantly different between AS and normal AA cells,47 others point out differences in RBC from SCT individuals which can adversely affect RBC processing and storage.7,48
In this study, we observed pronounced variability in both initial MF of RBC from SCT subjects and in cells’ response to hypoxia and follow-up reoxygenation. Inter- estingly, unlike AA samples, for AS samples the initial MF was a poor predictor of cells’ response to hypoxia and reoxygenation. As anticipated, for all samples hyp- oxia resulted in increased cell fragility, however, the scale of such increase was found to be highly variable. For example, comparing the response from subjects ‘‘1’’ and ‘‘2’’ per Fig 3, while similar initially, hypoxia induced nearly double the increase in RBC fragility for subject ‘‘2’’ as for subject ‘‘1.’’ Moreover, while upon exposure to oxygen, for subject ‘‘1’’ cell fragility reverted to close to its prehypoxia values (similar to the behavior of AA cells), membrane changes for subject ‘‘2’’ cells seemed to be irreversible. Such irreversible changes in MF are likely due to deep hypoxia-induced plastic/per- manent deformation of the membrane, possibly linked to irreversible cell sickling. We hypothesize that essen- tially irreversible changes in RBC MF upon a single deoxygenation-reoxygenation cycle could be indicative of an elevated risk of SCA-type clinical complications. If validated, the ability to recognize such high-risk indi- viduals through a simple MF test may be a useful tool in managing patients with SCT. Similarly, SCT donor blood can be tested, and when at risk of hypoxia-induced irre- versible RBC change, and packed RBC can be diverted from transfusion toward other use. Technology facili- tating RBC MF testing is under development.49
For SS cells, the present work found cell fragility to increase upon deoxygenation, and, on average, to decrease when oxygen was reintroduced. These results correlate with those reported previously.5,22 However,

RBC from SCA patients, similar to that from SCT individuals, exhibited significant heterogeneity in their ability to recover. Hypoxic insult on RBC from some subjects (eg, 6 or 8, Fig 4) induced irreversible (on an up to 1-hour time scale) change in cell MF, while RBC MF from other subjects (eg, 4 or 5, Fig 4) upon re- oxygenation reverted to its prehypoxia values (similar to AA cells). As with SCT subjects, irreversible change in MF could imply membrane damage due to plastic defor- mation, with or without associated irreversible sickling. In any case, such changes could potentially lead to increased Hem and higher risk of vaso-occlusion.
This observation of a heterogeneous response to deox- ygenation may possibly reflect clinical heterogeneity of SCD, the severity of which can vary wildly among pa- tients.50,51 In that regard, it had been suggested that genetically predisposed severity of the disease could be modulated by membrane alterations of sickle RBC.3 Naturally, the high variability in response of SS (and AS) cells to a cycle of deoxygenation involving severe hypoxic conditions observed in this work remains to be correlated with complication rates and severity. If vali- dated, it could offer a practical test (MF) indicative of which patients are more at risk for extensive pathology. If such were indeed the case, it would allow better- targeted and potentially timelier interventions.
The symptoms of SCA can be alleviated with HU which was shown to stimulate nitric oxide produc- tion—possibly through HU oxidation, its reaction with Hb, or enhanced ATP release from cells.52-54 The capacity of HU to increase Hb-F levels in RBC had also been suggested as one of the mechanisms, as Hb-F inhibits deoxy sickle Hb-S polymerization,55 making cells more likely to survive in circulation (eg, Steinberg et al56). Response to HU therapy is highly varied; while some patients show up to 40% increase in Hb-F concen- trations, others show only minor changes,56,57 with familial factors (genetic or environmental) potentially influencing Hb-F synthesis.58 While poor correlation be- tween Hb-F levels and severity of complications had been reported,59 Hb-F is still considered the most impor- tant and best characterized modifier of SCD severity, which at high concentrations is able to ameliorate most of the disease symptoms.39 (The variability in clinical ef- fects could be due to the heterogeneous distribution of Hb-F among individual erythrocytes. While cells high in Hb-F would be ‘‘protected,’’ cells with low Hb-F con- tent would have higher propensity to sickle, giving rise to a progression of events resulting in more severe SCA.56) Thus, reactivation of Hb-F production, based on reversing of BCL11A repressor, may be a focus of devel- opment of potential new therapies for SCA.60
In this work, we did not observe a correlation between HU dose and blood Hb-F concentration; however, patien
compliance with the therapy was not verified. Contrary to expectations, Hb-F cell concentration was not negatively correlated with an increase in RBC MF; but it did corre- late with cell recovery when oxygen was reintroduced. However, unlike gender, it did not speak to how close the resultant fragility would be to its original (prehy- poxia) values. Some individuals (eg, subjects 4 or 5, with high Hb-F concentration) exhibited good recovery, while others (eg, subjects 6 or 9, with low Hb-F) showed essentially none. Higher Hb-F concentrations have been associated previously with decreased irreversible sick- ling,61 possibly due to a low fraction of irreversible bind- ing of deoxygenated Hb-S with the cdb3 complex.
There are a number of limitations to this pilot study. In some cases, as stipulated in the text, sample sizes were too small to draw statistical inference with suffi- cient power. Moreover, results indicated a possibility of subgroups (Table II) in all subject groups; however, subgroup analysis would require larger sample sizes, with recruitment tailored to such subgroup analysis. As mentioned, compliance with HU therapy was not evaluated, and thus, any inference of its possible corre- lation with Hb-F or RBC MF carries additional uncer- tainty. Levels of hypoxia used in this work are likely more severe than physiological, which likely resulted in RBC MF changes more severe that those observed clinically. (Equilibration time for both Hb deoxygen- ation and reoxygenation are orders of magnitude longer than that reported for typical time in cell transit when it may be subjected to low oxygen conditions, as well as time associated with the either Hb-S polymerization delay or polymerized Hb-S melting.) However, MF changes observed at more severe (even if non- physiological) hypoxia conditions, if correlated with outcomes, could still have clinical significance as a diagnostic/monitoring test.
In summary, the presented results show that a single hypoxic event and cell recovery after such event can vary significantly among SS and SA patients, indicating variable amounts of induced irreversible (plastic) as opposed to reversible (elastic) cell deformations. These results could be useful for planning future experiments and sample size calculations based on estimates for mean and SDs of RBC MF under impact of hypoxia and reoxygenation, with an aim of validating ap- proaches for monitoring/evaluation of extended pathol- ogy risks for SCD patients.
ACKNOWLEDGMENTS
Conflicts of Interest: The authors Tarasev, Muchnik, Alfano, and Chakraborty are employed and/or have eq- uity with Blaze Medical Devices, with Light having no conflict of interest to disclose. The authors have read the

journal’s authorship agreement and policy on disclosure of potential conflicts of interest, and have reviewed and approved the manuscript.
This work was funded by Blaze Medical Devices which develops tests for measuring erythrocyte fragility. The authors thank Dr Abba Zubair of Mayo Clinic, Jacksonville, for helpful suggestions regarding study design; also gratefully acknowledged are personnel of BloodCenter of Wisconsin, particularly Monika de Ar- ruda Indig and Sharon Graminske for helpful comments, subject recruitment, and study coordination.
REFERENCES
- Disease and conditions index. Sickle cell anemia: who is at risk? [Internet]. Bethesda, MD: US Department of Health and Human Services, National Institutes of Health, National Heart, Lung, and Blood Institute. Available at: http://www.nhlbi.nih.gov/ health/health-topics/topics/sca/; 2012. Accessed October 12, 2016.
- Eaton JW, Jacob HS, White JG. Membrane abnormalities of irre- versibly sickled cells. Semin Hematol 1979;16:52–64.
- Hebbel RP. Beyond hemoglobin polymerization: the red blood cell membrane and sickle disease pathophysiology. Blood 1991; 77:214–37.
- Barodka VM, Nagababu E, Mohanty JG, et al. New insights pro- vided by a comparison of impaired deformability with erythrocyte oxidative stress for sickle cell disease. Blood Cells Mol Dis 2014; 52:230–5.
- MacCallum RN, Lynch EC, Hellums JD, et al. Fragility of abnormal erythrocytes evaluated by response to shear stress. J Lab Clin Med 1975;85:67–74.
- Barbedo MM, McCurdy PR. Red cell life span in sickle cell trait. Acta Haematol 1974;51:339–43.
- Stroncek DF, Rainer T, Sharon V, et al. Sickle Hb polymerization in RBC components from donors with sickle cell trait prevents effective WBC reduction by filtration. Transfusion 2002;42: 1466–72.
- Lawrie AS, Pizzey A, Trompeter S, et al. Procoagulant activity in patients with sickle cell trait. Blood Coagul Fibrinolysis 2012;23: 268–70.
- Chasis JA, Mohandas N. Erythrocyte membrane deformability and stability: two distinct membrane properties that are indepen- dently regulated by skeletal protein associations. J Cell Biol 1986; 103:343–50.
- Clark MR, Mohandas N, Shohet SB. Deformability of oxygenated irreversibly sickled cells. J Clin Invest 1980;65:189–96.
- Noguchi CT, Schechter AN. Non-uniformity of intracellular poly- mer formation in sickle erythrocytes: possible correlation with severity of hemolytic anemia. Am J Pediatr Hematol Oncol 1984;6:46–50.
- Green MA, Noguchi CT, Keidan AJ, et al. Polymerization of sickle cell hemoglobin at arterial oxygen saturation impairs eryth- rocyte deformability. J Clin Invest 1988;81:1669–74.
- Shaklai N, Ranney HM, Sharma V. Interactions of hemoglobin S with the red cell membrane. Prog Clin Biol Res 1981;51: 1–16.
- Nash GB, Johnson CS, Meiselman HJ. Influence of oxygen ten- sion on the viscoelastic behavior of red blood cells in sickle cell disease. Blood 1986;67:110–8. Alapan Y, Little JA, Gurkan UA. Heterogeneous red blood cell adhesion and deformability in sickle cell disease. Sci Rep 2014; 4:7173
- Ballas SK, Connes P. The paradox of the serrated sickle erythro- cyte: the importance of the red blood cell membrane topography. Clin Hemorheol Microcirc 2015;63:149–52.
- Alvarez O, Montague NS, Marin M, et al. Quantification of sickle cells in the peripheral smear as a marker of disease severity. Fetal Pediatr Pathol 2015;34:149–54.
- Reiter CD, Wang X, Tanus-Santos JE, et al. Cell-free hemoglobin limits nitric oxide bioavailability in sickle-cell disease. Nat Med 2002;8:1383–9.
- Jeney V, Balla J, Yachie A, et al. Pro-oxidant and cytotoxic effects of circulating heme. Blood 2002;100:879–87.
- Kato GJ, Gladwin MT, Steinberg MH. Deconstructing sickle cell disease: reappraisal of the role of hemolysis in the development of clinical subphenotypes. Blood Rev 2007;21:37–47.
- Nouraie M, Lee JS, Zhang Y, et al. The relationship between the severity of hemolysis, clinical manifestations and risk of death in 415 patients with sickle cell anemia in the US and Europe. Hae- matologica 2013;98:464–72.
- Presley TD, Perlegas AS, Bain LE, et al. Effects of a single sick- ling event on the mechanical fragility of sickle cell trait erythro- cytes. Hemoglobin 2010;34:24–36.
- Tarasev M, Alfano K, Chakraborty S, et al. Similar donors-similar blood? Transfusion (Paris) 2014;54:933–41.
- Nash GB, Johnson CS, Meiselman HJ. Mechanical properties of oxygenated red blood cells in sickle cell (HbSS) disease. Blood 1984;63:73–82.
- Sowemimo-Coker SO. Red blood cell hemolysis during process- ing. Transfus Med Rev 2002;16:46–60.
- Prahl S. Optical absorption of hemoglobin [Internet]. Portland, OR: Oregon Optical Laser Center. Available at: http://omlc.org/ spectra/hemoglobin/summary.html; 1999. Accessed October 12, 2016.
- van Assendelft OW, Zijlstra WG. Extinction coefficients for use in equations for the spectrophotometric analysis of haemoglobin mixtures. Anal Biochem 1975;69:43–8.
- Zijlstra WG, Buursma A. Spectrophotometry of hemoglobin: ab- sorption spectra of bovine oxyhemoglobin, deoxyhemoglobin, carboxyhemoglobin and methemoglobin. Comp Biochem Physiol 1997;118B:743–9.
- Raval JS, Waters JH, Seltsam A, et al. The use of the mechanical fragility test in evaluating sublethal RBC injury during storage. Vox Sang 2010;99:325–31.
- Alfano K, Tarasev M. Investigating direct non-age metrics of stored blood quality loss. Internet J Med Tech 2011;5.
- Stefanovic M, Puchulu-Campanella E, Kodippili G, et al. Oxygen regulates the band 3-ankyrin bridge in the human erythrocyte membrane. Biochem J 2013;449:143–50.
- Hakim TS, Macek AS. Effect of hypoxia on erythrocyte deform- ability in different species. Biorheology 1988;25:857–68.
- Uyuklu M, Meiselman HJ, Baskurt OK. Effect of hemoglobin oxygenation level on red blood cell deformability and aggregation parameters. Clin Hemorheol Microcirc 2009;41:179–88.
- Maha AA. Effect of glucose-6-phosphate dehydrogenase defi- ciency on some biophysical properties of human erythrocytes. He- matology 2009;14:38–45.
- Soranzo N, Spector TD, Mangino M, et al. A genome-wide meta- analysis identifies 22 loci associated with eight hematological pa- rameters in the HaemGen consortium. Nat Genet 2009;41: 1182–90.
- Capuano P, Catalano G, Garruti G, et al. The effects of weight loss due to gastric banding and lifestyle modification on red blood ceaggregation and deformability in severe obese subjects. Int J Obes (Lond) 2012;36:342–7.

- Senturk UK, Gunduz F, Kuru O, et al. Exercise-induced oxidative stress leads hemolysis in sedentary but not trained humans. J Appl Physiol 2005;99:1434–41.
- Kilic-Toprak E, Ardic F, Erken G, et al. Hemorheological re- sponses to progressive resistance exercise training in healthy young males. Med Sci Monit 2012;18:CR351–60.
- Quinn CT. Minireview: clinical severity in sickle cell disease: the challenges of definition and prognostication. Exp Biol Med (May- wood) 2016;241:679–88.
- Liu SC, Derick LH, Zhai S, et al. Uncoupling of the spectrin-based skeleton from the lipid bilayer in sickled red cells. Science 1991; 252:574–6.
- Franck PF, Bevers EM, Lubin BH, et al. Uncoupling of the mem- brane skeleton from the lipid bilayer. The cause of accelerated phospholipid flip-flop leading to an enhanced procoagulant activ- ity of sickled cells. J Clin Invest 1985;75:183–90.
- van Beers EJ, Schaap MC, Berckmans RJ, et al. Circulating erythrocyte-derived microparticles are associated with coagulation activation in sickle cell disease. Haematologica 2009;94:1513–9.
- Tsaras G, Owusu-Ansah A, Boateng FO, et al. Complications associated with sickle cell trait: a brief narrative review. Am J Med 2009;122:507–12.
- Kark JA, Posey DM, Schumacher HR, et al. Sickle-cell trait as a risk factor for sudden death in physical training. N Engl J Med 1987;317:781–7.
- Naik RP, Haywood C Jr. Sickle cell trait diagnosis: clinical and so- cial implications. Hematology Am Soc Hematol Educ Program 2015;2015:160–7.
- Noguchi CT, Torchia DA, Schechter AN. Polymerization of he- moglobin in sickle trait erythrocytes and lysates. J Biol Chem 1981;256:4168–71.
- Adewuyi JO, Awarun JA. Deformability of stored normal and sickle haemoglobin erythrocytes. Afr J Med Med Sci 1990;19: 115–9.
- Brandao MM, Saad ST, Cezar CL, et al. Elastic properties of stored red blood cells from sickle trait donor units. Vox Sang 2003;85:213–5 Alfano KM, Tarasev M, Meines S, et al. An approach to measuring RBC haemolysis and profiling RBC mechanical fragility. J Med Eng Technol 2016;40:162–71.
- Steinberg MH. Predicting clinical severity in sickle cell anaemia. Br J Haematol 2005;129:465–81.
- Alli NA, Patel M, Alli HD, et al. Recommendations for the man- agement of sickle cell disease in South Africa. S Afr Med J 2014; 104:743–51.
- Huang J, Yakubu M, Kim-Shapiro DB, et al. Rat liver-mediated metabolism of hydroxyurea to nitric oxide. Free Radic Biol Med 2006;40:1675–81.
- Lockamy VL, Shields H, Kim-Shapiro DB, et al. Iron nitrosyl he- moglobin formation from the reaction of hydroxylamine and he- moglobin under physiological conditions. Biochim Biophys Acta 2004;1674:260–7.
- Lockwood SY, Erkal JL, Spence DM. Endothelium-derived nitric oxide production is increased by ATP released from red blood cells incubated with hydroxyurea. Nitric Oxide 2014;38:1–7.
- Platt OS, Orkin SH, Dover G, et al. Hydroxyurea enhances fetal hemoglobin production in sickle cell anemia. J Clin Invest 1984;74:652–6.
- Steinberg MH, Chui DH, Dover GJ, et al. Fetal hemoglobin in sickle cell anemia: a glass half full? Blood 2014;123:481–5.
- Pule GD, Mowla S, Novitzky N, et al. A systematic review of known mechanisms of hydroxyurea-induced fetal hemoglobin for treatment of sickle cell disease. Expert Rev Hematol 2015;8: 669–79.
- McCormack MK, Bresson VL, Serjeant GR. Letter: fetal hemo- globin and the irreversibly sickled cell in sickle cell disease. J Pe- diatr 1974;85:435–6.
- Seltzer WK, Abshire TC, Lane PA, et al. Molecular genetic studies in black families with sickle cell anemia and unusually high levels of fetal hemoglobin. Hemoglobin 1992;16:363–77.
- Xu J, Peng C, Sankaran VG, et al. Correction of sickle cell disease in adult mice by interference with fetal hemoglobin silencing. Sci- ence 2011;334:993–6.
- Bertles JF, Milner PF. Irreversibly sickled erythrocytes: a conse- quence of the heterogeneous distribution of hemoglobin types in sickle-cell anemia. J Clin Invest 1968;47:1731–41.