MILITARY MEDICINE, 180, 3:150, 2015
RBC Mechanical Fragility as a Direct Blood Quality Metric to Supplement Storage Time
Michael Tarasev, PhD, MBA; Sumita Chakraborty, PhD; Kenneth Alfano, MS, JD
ABSTRACT Introduction: Lengthy storage times and associated storage lesion can result in reduced red blood cell (RBC) efficacy, particularly dangerous for massively transfused patients. Today’s inventory management makes storage times the de-facto metric of blood quality. However, RBC units’ quality may vary because of time-independent factors. Mechanical fragility (MF) of RBC, reflecting sub-lethal cell damage, can potentially provide a more physiologically relevant predictor of cell’s performance “in vivo.” Methods: Mechanical stress was applied using a bead mill (50 Hz) over durations varying from 0.5 to 60 minutes, or using ultrasound (40 W) with durations from 0.1 to 120 seconds. MF profiles were described in terms of percentage hemolysis following stresses of specified durations. Results: RBC MF declined significantly in the presence of albumin, with albumin protecting membrane against damage from elevated temperature or from methyl-b-cyclodextrin or diamide. MF profiles allowed detection of sub-lethal membrane damage caused by elevated temperature, to a greater extent than was reflected by autohemolysis. Different types of profiles for RBC damage were associated with MF changes at different stress intensities and potentially stress types. Conclusions: These findings indicate that MF profiles can provide a powerful and versatile tool for investigation of RBC, as well as a potential metric of RBC quality.
INTRODUCTION
Modern warfare causes severe injuries with frequently severe blood loss. Massive transfusions of up to 10 to 15 units per person are often required to stabilize such patients. Severe trauma patients, typical for military hospitals and battlefield care, also may require higher blood hemoglobin levels in addition to volume replacement and perfusion. In those cases, tissue oxygen supply may be additionally constrained by increased metabolic requirements, arterial hypoxemia, or alka- losis. This is at times combined with limitations on medical monitoring and availability of critical support measures like ventilators.1,2 Thus, the efficacy of each transfused blood unit, understood as ability of red blood cells (RBC) to survive in the bloodstream and deliver oxygen to the tissues, becomes a subject of utmost concern.
Packed Red Blood Cells (pRBC) is the most commonly transfused blood component ordinarily supplied by the Armed Services Blood Program, from donations collected and proc- essed in the United States and transported overseas to military medical facilities. Lengthy storage times are often logistically- necessitated, and blood units can be 27 to 32 days old on delivery and 32 days or older at the time of transfusion, compared to the 21-day average storage time (ST) for trans- fused units in civilian U.S. hospitals.3
RBC storage is associated with cell deterioration (storage lesion), and its relevance to clinical outcomes for various patients and diagnosis-related groups is a subject of much debate.4–7 Severe trauma seems to present an exceptionally
Blaze Medical Devices, 330 East Liberty Street, Lower Level, Ann Arbor, MI 48104.
This article was presented at the Military Health System Research Sym- posium, Fort Lauderdale, FL, August 12–15, 2013.
doi: 10.7205/MILMED-D-14-00404
high challenge for the quality of blood products used, an observation supported by multiple studies looking at the effects of storage lesion on transfusion outcomes for that patient group.8–12
Significant effort is exerted to determine whether “new” blood provides better outcomes than “old” blood—with ABLE,13 RECESS,14 ARIPI,15,16 and other studies aiming to answer that question. This focus on the RBC ST as essen- tially the sole quality metric is also exemplified by the com- mon inventory management approach focused on using the oldest blood first. This approach implicitly assumes equal “quality” or potential efficacy of units of the same age, which is likely a false assumption in light of significant unit-to-unit variability.17,18 That interunit variability could be a result of inherent donor-to-donor variability in cells’ biochemical and biomechanical properties including the cell metabolism,19 metabolic age,20 and initial ATP levels.21 Variability in donor- related RBC properties could be further amplified through manufacturing, transportation and storage.22
Mechanical fragility (MF) of the RBC is a quantifiable property that shows potential to provide a supplemental or alternative measure of blood quality. Spontaneous hemolysis or “autolysis” has long been investigated and is already a part of current quality standards (albeit at an aggregate level), but the measured ability of RBC to withstand applied mechanical stress is a more comprehensive reflection of existing sub-lethal cell damage, and better resembles the cells’ survival and performance “in vivo” posttransfusion. MF23,24 and related flow properties25,26 have been proposed as more physiologic candidates to supplement ST as an aggregate metric of RBCs’ functional and structural storage lesions. It was hypothesized that MF could serve as an aggregate metric of cells’ health and, indirectly, of changes in the cells’ environment (e.g., free hemoglobin [Hb], microvesicles, proinflammatory cytokines)
MILITARY MEDICINE, Vol. 180, March Supplement 2015
Downloaded from publications.amsus.org: AMSUS - Association of Military Surgeons of the U.S. IP: 141.214.017.252 on Mar 10, 2015. Copyright (c) Association of Military Surgeons of the U.S. All rights reserved.
which could be linked with membrane-related changes (up to and including autohemolysis [AH]). We had previously dem- onstrated significant variability of RBC MF properties in units at the point of use, which was largely independent of ST, potentially depended on the method of unit manufactur- ing, and was largely attributed to donor-to-donor differ- ences.27 Significant unit-to-unit differences were observed even between units drawn from similar donors, with such units varying both in their MF at the time of collection and in their rates of change of MF over Although clinical relevance of the observed differences in MF among units remains to be demonstrated in clinical stud- ies, further exploration of MF as a potential metric of RBC “quality” seems warranted. This article presents methods for comprehensive assessment of RBC MF, which appears sensi-tive enough to reflect RBC MF profile complexity that in turn reflects multiple factors influencing MF, and addresses poten- tial approaches for implementation in clinical practice.
MATERIALS AND METHODS
Mechanical Fragility Test
RBC samples, obtained from leukoreduced nonirradiated packed cells in AS3 storage solution, were sedimented by centrifuging for 5 minutes at 5,000 rpm on an Eppendorf 5417C Centrifuge (Hamburg, Germany); supernatant was removed and replaced with an equal volume of AS3, pH 5.75 and supplemented where indicated with 4 g/dL albumin. Before stress application, samples were further diluted to a total hemoglobin concentration of 1.7 g/dL (corresponding to about 4% hematocrit) with the same solutions as above. For stress application using a bead mill, the diluted sample was gently agitated and aliquoted into 2 mL low-retention centri- fuge tubes at 350 mL per tube. Mechanical stress was applied to pRBC samples with the use of a TissueLyser LT (Qiagen, Dusseldorf, Germany) bead mill at an oscillation frequency of 50 Hz, in the presence of one 7-mm-diameter stainless steel ball, for a predetermined duration. Samples from each pRBC unit were subjected to such stress at 10 different dura- tions (ranging from 30 seconds to 60 minutes) to ensure a wide range of achieved hemolysis levels. The sample holder of the TissueLyser was modified to allow air cooling while in operation, which resulted in sample temperature stabilization to within 2oC of the start temperature. Unlysed cells were sedimented by centrifuging the samples for 5 minutes at 5,000 rpm. Supernatant samples were collected and used for spectral analysis. For stress application using ultrasound, 6 mL of diluted pRBC sample was sonicated using Branson (Danbury, Connecticut) Digital Sonifier 450, at 10% intensity (from the manufacturer specified 400 W), with durations rang- ing from 0.1 second to 2 minutes, with sonication application in 0.1-second pulses with 0.5-second intervals in-between pulses, in a water bath. Aliquots (30–50 mL) were taken and processed as above to measure hemolysis at set durations.
Hemolysis Assessment
Hemolysis (Hem), in both unstressed sample (such hemolysis being AH) and as that stress induced by the bead mill, was determined based on the difference in absorbance at 576 nm, a wavelength of oxygenated Hb maximum, and absorbance at 700 nm. It was expressed as a fraction of free hemoglobin (HbF) relative to total hemoglobin concentration (HbT) accord- ing to Formula 1, which included the correction for sample hematocrit as detailed by Sowemino-Coker.29
Total hemoglobin concentration for each diluted pRBC sample was determined by subjecting a small (30–40 mL aliquot to repeated (5) rapid freeze-thaw using liquid nitro-gen. In control experiments, such treatment was shown to fully lyse RBC. For AH (hemolysis before the application of mechanical stress) determination, small (20 mL) samples of undiluted segments’ content were centrifuged for 5 minutes at 1,300 rpm, supernatants were collected, and hemoglobin content was measured spectrophotometrically. Spectroscopic measurements were performed with a NanoDrop N100 spec- trophotometer (NanoDrop, Thermo Scientific).
RBC Mechanical Fragility Profiles
These are defined here as the cumulative incremental (beyond AH) hemolysis resulting from applied stress of vary- ing durations. Unlike single-point measurements that use a single stress duration at a single stress intensity, as imple- mented, for example, by Raval et al,24 MF profiles allow ascertainment of the propensity for red cells to hemolyse “over a range” of applied stress magnitudes. These include levels of duration and/or intensity resulting in minimal induced hemo- lysis, ranging up to those resulting in nearly total hemolysis of cells in the tested sample—thereby allowing multiple fragility- based indexes to be interpolated for separate analyses.23 Where required, profiles were described by the hemolysis parameters representing the extent of hemolysis (Hem, in percent hemolysis) achieved as a result of the stress duration indicated in the subscript. Each data point is the average of three independent measurements, with average standard devi- ation of 8%, unless specified otherwise. Fragility parameters were obtained from best-fit second-order polynomial regres- sion to the experimental data. For curves exhibiting signifi- cant deviations from a simple polynomial, raw data were subdivided into low and high hemolysis subsets and the fits were obtained independently for each subset of the data.
Effect of Storage Time
Under institutional review board approval, 13 paid research donors between the ages of 24 and 59 were recruited and consented to participate. All donors were male, nonsmoking, and of the same blood type (A+) and eligible to donate under
MILITARY MEDICINE, Vol. 180, March Supplement 2015
Downloaded from publications.amsus.org: AMSUS - Association of Military Surgeons of the U.S. IP: 141.214.017.252 on Mar 10, 2015. Copyright (c) Association of Military Surgeons of the U.S. All rights reserved.
current Red Cross standards. Nonleukoreduced pRBCs were prepared per standard protocol from each whole blood unit collected by centrifugation. pRBCs were stored at 4oC to 6oC and sterilely sampled at 4, 14, 28, and 42 days postcollection.
Materials
pRBC samples were received from the University of Michigan Hospital Blood Bank and Memorial Blood Centers (Minnesota); samples of fresh frozen plasma were obtained from the Red Cross of Michigan. Albumin was from RPI (Mount Prospect, Illinois); all other materials were from Sigma-Aldrich (St. Louis, Missouri).
RESULTS
Mechanical Fragility Profiles of Normal and Hardened RBC When stressed via a bead mill in AS3 storage solution, pro- gressively increasing durations of stress induced progres- sively increasing levels of hemolysis of RBC. Sampling of resulting hemolysis, as reflected by changes in cell-free Hb, over the duration of applied stress allows generating MF profiles of the sample, as shown in Figure 1. RBC MF can be significantly affected by cells’ environ- ment, as evidenced by marked changes in recorded MF pro- files when the media is supplemented with a physiological concentration of albumin (Fig. 1). Supplementation of AS3
with equimolar concentrations of PEG 4,000 and PEG 20,000 resulted in a similar, but much less pronounced (by about a third to a half) decrease in RBC MF (data not shown). This observation indicates that while viscosity and/or osmolality changes do play a role in the apparent decrease in RBC MF in the presence of albumin, a substantial part of the effect is likely because of protein/membrane interactions.
Sensitivity of the proposed methods of assessing RBC MF can be evaluated through the use of chemical compounds known to affect the rigidity and/or deformability of RBC membrane. In particular, diamide (DA) was shown to increase the shear modulus and viscosity of the RBC membrane skel- eton by creating disulphide bonds preferentially on the spectrin protein, thus potentially resulting in a more rigid and less deformable membrane.30 Cyclodextrins and methyl- b-cyclodextrin (MCD) in particular were reported to also affect RBC membrane, likely through the extraction of cho- lesterol, also reducing cell deformability.31 After incubation with DA or MCD, minimal changes in RBC morphology were observed, with cells predominantly retaining their orig- inal discoid shape. However, incubation with either drug resulted in pronounced changes to cells’ mechanical fragility. In particular, for cells suspended in AS3 supplemented with albumin at physiological concentration, incubation resulted in cells with higher fragility, when tested with stress applied via a bead mill (Fig. 1). MF was strongly dependent on the concentration of DA and MCD in the solution, with the MF changes clearly well resolvable at concentrations two orders
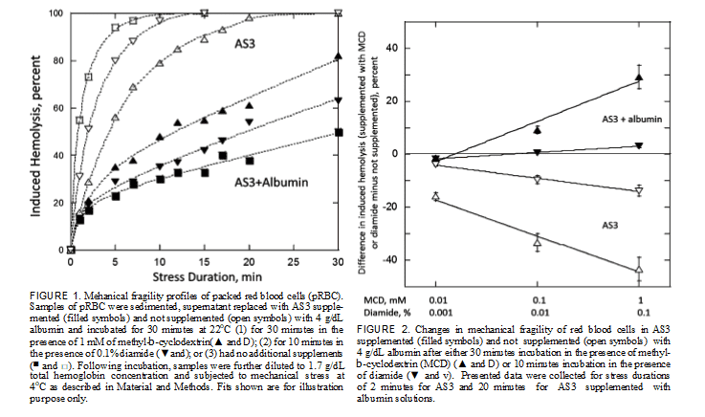
MILITARY MEDICINE, Vol. 180, March Supplement 2015
Downloaded from publications.amsus.org: AMSUS - Association of Military Surgeons of the U.S. IP: 141.214.017.252 on Mar 10, 2015. Copyright (c) Association of Military Surgeons of the U.S. All rights reserved.
of magnitude below those that are commonly used in RBC membrane studies (i.e., 0.1% for DA and 1 mM for MCD) (Fig. 2). Paradoxically, when albumin was not present, the same incubation resulted in cells better able to withstand mechanical stress (as applied via a bead mill). This effect was also concentration dependent (Fig. 2). It seems that drug-induced changes in RBC membrane that, as reported, resulted in cells’ loss of deformability made them also less susceptible to hemolysis. Albumin by itself significantly increased resistance to hemolysis (decreased MF), but its effect was seemingly counteracted by the drugs, which could have disrupted its interaction with cell membrane. When potentially lethal external stress was applied using ultrasound, only minimal changes (under 5%) were observed
on supplementation of AS3 storage media with albumin. Similarly, incubation with DA (up to 0.1%) or MCD (up to 1 mM) effected no changes in RBC propensity to hemolyse, with no changes to RBC MF profiles detected irrespective of whether the media was supplemented with albumin or not (data not shown).
Effect of Temperature on RBC MF
pRBC are stored at 4oC, with the use of blood warming devices recommended for massively transfused patients to prevent hypothermia. That raises the question of stability of pRBC when exposed to elevated, as compared to storage, tempera- tures. AH in pRBC samples (sedimented, with supernatant replaced with AS3 supplemented with albumin) gradually
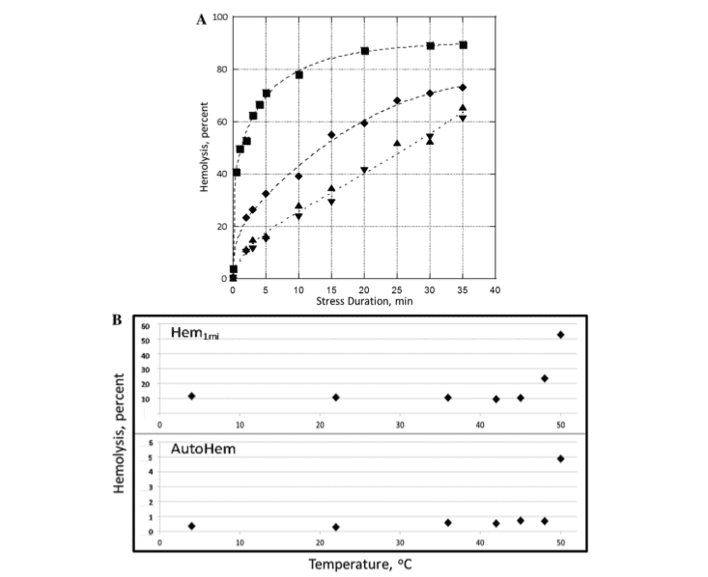
FIGURE 3. Mechanical fragility of packed red blood cells suspended in AS3 supplemented with 4 g/dL albumin. (A): Mechanical fragility profiles after 30 minutes incubation at 4oC (▲), 45oC (▼), 48oC (¨), and 50oC (▪). (B): Autohemolysis (Hem0) and 1 minute of induced hemolysis at 30-Hz oscillation frequency (Hem1) over the range of temperatures from 4oC to 50oC. Fits shown are for illustration purpose only
MILITARY MEDICINE, Vol. 180, March Supplement 2015
Downloaded from publications.amsus.org: AMSUS - Association of Military Surgeons of the U.S. IP: 141.214.017.252 on Mar 10, 2015. Copyright (c) Association of Military Surgeons of the U.S. All rights reserved.
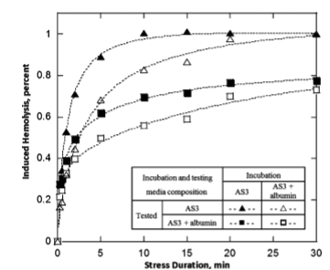
FIGURE 4. Mechanical fragility of packed red blood cells incubated for 30 minutes at 47oC, incubated with and without supplementing albumin. Samples were washed with AS3 postincubation with the buffer replaced with either AS3 or AS3 supplemented with albumin. See graph for details. Fits shown are for illustration purpose only.
increased from about 0.3% at 4 and 22oC to about 0.7% at 45oC to 48oC. Further temperature increase to 50oC resulted in a discontinuous increase in AH to about 5% (Fig. 3A). RBC MF as tested via bead milling remained essentially unchanged following incubation at temperatures in the range from 4oC to 45oC. Notably, for MF a marked increase was observed already at 48oC, with cells becoming even more fragile at 50oC (Fig. 3B). Overall, the scope of response was more significant for MF than for AH (Hem0)-indicating that lysis (cell lethal damage) represents only a small subset of cells that suffered thermally induced membrane damage. Samples incubated at elevated temperature in AS3 solu- tion that was not supplemented with albumin exhibited higher propensity for hemolysis than those in the presence of albumin (Fig. 4). Cells that were both incubated and tested in the presence of albumin had the lowest MF, whereas the cells with no albumin present either at incubation or at testing had the highest. Thus, albumin not only enhanced RBCs’ ability to withstand mechanical stress during the application of stress
but also partially protected RBC from high temperature- induced damage.
Effect of Storage
We previously reported the results of monitoring the changes in RBC MF through storage with stress applied using a bead mill.28 The values for MF determined that way, as well as the magnitude of AH, varied significantly at collection as well as exhibited pronounced variability in the rates of change over the storage time. MF as determined with stress applied by ultrasound also had high unit-to-unit variability (Table I). No significant correlation between the MF via ultrasound and MF via bead mill was detected (which is consistent with others’ observations, noted in the discussion below).
For both ultrasound-applied and bead-mill-applied stress, significant variability across donors in both the Hem parame- ters at 5 days postcollection and in the rates of change was observed (see Table I and Fig. 5). When stress was applied using the bead mill, the average MF values increased over the ST, with such changes being progressively less pronounced for those MF parameters involving higher overall stress mag- nitude (Fig. 5; Hem parameters for 1 and 30 minute stress). Statistically, relatively small observed ST-dependent changes in Hem30min values were not significantly different from 0, whereas those for Hem1min were well pronounced (Table I). For ultrasound also, when probed by short (e.g., 1 second in duration) stress, most of the units exhibited higher hemolysis with increased duration of storage; however, with larger (e.g., 6 seconds in duration) stress used, the magnitude of induced hemolysis and thus of RBC MF actually decreased over the storage time.
“Donor 5’s” unit was identified previously as that derived from a donor with undisclosed hereditary hyperlipidemia and a triglyceride level at time of donation of 1,056 mg/dL.28 When tested using ultrasound, changes over the ST in the MF of “donor 5’s” RBC less-obviously deviated from that of the other units. It should be noted, that for MF by both bead-mill-induced and ultrasound-induced stress, significant donor-to-donor variability was observed (Fig. 5). Thus, while the fragility of some units appeared to decrease, that of others was actually increasing, thus resulting in the observed high variability among the rates of change. Similar inconsistent behavior when applying MF stress using ultrasound was
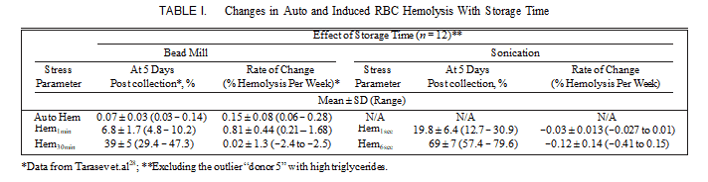
MILITARY MEDICINE, Vol. 180, March Supplement 2015
Downloaded from publications.amsus.org: AMSUS - Association of Military Surgeons of the U.S. IP: 141.214.017.252 on Mar 10, 2015. Copyright (c) Association of Military Surgeons of the U.S. All rights reserved.
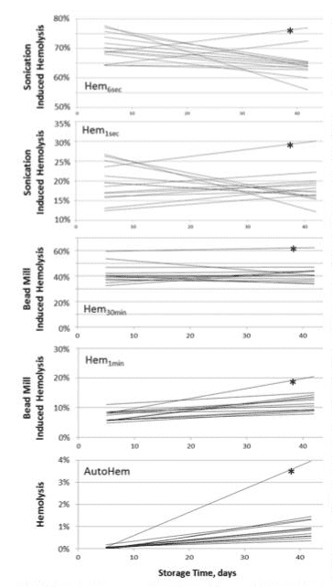
FIGURE 5. Changes in auto (AutoHem) and induced hemolysis as a function of storage time. Presented are best-fit linear trend lines for the experimental data from the 13 donors measured at 5, 14, 28, and 42 days postblood collection. Stress was applied using a bead mill (Hem1min and Hem30min) and by sonication (Hem1sec and Hem6sec). Asterisks mark trend lines calculated from the “donor 5” data.
described previously.32 Although a similar effect was noted in the case when bead mill was used, particularly for longer stress applications, it was substantially less pronounced than in cases of ultrasonic stress. The mechanisms responsible for such diverse behavior remain to be elucidated in future research.
DISCUSSION
Erythrocyte mechanical fragility testing pertains to a notion of cells’ propensity for or susceptibility to hemolysis under
mechanical stress. It has the potential to more directly reflect erythrocyte membrane properties, compared for example to osmotic fragility, which would also depend on nonme- chanical aspects such as intracellular ion concentration and transmembrane water/ion transfer rates (in addition to the mechanical properties).
Approaches to providing mechanical stress can be grouped into two broad categories: “high-energy” mechanical stress, and “low-energy” mechanical stress. Low-energy mechanical fragility, such as that utilizing a bead mill, tends to more directly reflect erythrocyte membrane properties, whereas high-energy mechanical fragility, such as that utilizing soni- cation for the stress, tends to more directly reflect hemoglo- bin viscosity and cell size.32 Thus, changes in cell membrane properties, as occur in RBC storage, would be reflected in RBC MF when tested with a bead mill. On the other hand, such changes would have only marginal effect on RBC MF when tested using ultrasound. However, prolonged storage results in decreased RBC cell size and increased hemoglobin viscosity, which would tend to decrease RBC MF as tested by ultrasound fragility. This explains the disparate results of pRBC MF changes in storage when probed by low energy (bead mill) and high energy (ultrasound) methods, noting too that the relationship between stress “intensity” (e.g., bead milling force/frequency levels) and stressing “energy” (e.g., bead milling vs. sonication approaches) remains to be fully elucidated. Interestingly, not only do the results of MF changes in RBC storage differ depending by whether they were measured using the bead mill or ultrasound but there are also qualitative differences in response at different stress durations even at a given stress intensity and type (i.e., total applied stress magnitude). Such differentiated responses may allow for development of diverse MF-based metrics corre- lated with different aspects of cell response to external mechanical stress.
When mechanical stress was applied using ultrasound, no difference was observed on RBC incubation with MCD or DA. Such incubation would modify RBC membrane struc- tures. At the same time, it did not induce any noticeable changes in cell morphology, with cell volumes and intracel- lular viscosity likely unchanged. Similarly to the previous observation, while low energy stress approaches could be expected to show changes in mechanical fragility, fragility as determined using high energy stress would be expected to remain unaffected. This effect was indeed observed experi- mentally (see Results). Significant variability in changes of pRBC MF during storage show that although some units experience degradation faster, others may be better able to withstand prolonged stor- age. This introduces the possibility of establishing a unit- specific RBC quality test to replace or supplement mere ST as an indicator of storage lesion—as well as rates thereof— thereby allowing modifications in today’s predominantly “first-in-first-out” (also known as “oldest out first”) inventory management practices. Additionally, it raises the prospect of
MILITARY MEDICINE, Vol. 180, March Supplement 2015
Downloaded from publications.amsus.org: AMSUS - Association of Military Surgeons of the U.S. IP: 141.214.017.252 on Mar 10, 2015. Copyright (c) Association of Military Surgeons of the U.S. All rights reserved.
potentially even extending the allowable shelf life for select units, based on the measurable preservation of their physio- logical properties—and ultimately of their prospective utility “in vivo.” Such uses could be especially helpful in military contexts, where long STs exacerbate the uncertainty of qual- ity caused by unit-to-unit variability. Alternatively, it may be possible to identify donors with comparatively “poorly stor- able” RBC, and use this information in guiding donor recruit- ment and attraction decisions, as well as initial routing of collected pRBC units. Other related applications could include triaging the most viable units for the most critical patients, or even tailoring selection of units for particular patients or patient groups based on particular RBC MF parameter(s) deemed specifically indicative of suitability for such patients—in the event that different MF-based metrics are found to reflect qualitatively different kinds of quality (e.g., relating to immediate efficacy vs. sustained efficacy).
Rapid transfusion in resuscitation could cause clinically dangerous hypothermia if unmodified, ice-cold blood were to be transfused. Thus, blood warmers may be used, with the requirement that blood must not be warmed above 42oC, or above a Food and Drug Administration approved manufac- turer’s recommendation. Prolonged exposure at room tem- perature33 or even up to 45oC in a blood warmer34 seem not to noticeably damage RBC, whereas rapid hemolysis accom- panied with severe morphological changes were reported to occur at temperatures over 45oC.35 Results presented above indicate that AH may be an insufficient indicator of cell degradation. Noticeable changes to cell membranes can occur, and be detectable through the use of MF profiles, without necessarily manifesting in elevated AH levels.
The ability of albumin to interact with cells’ plasma mem- branes, and with RBC membranes in particular, is well rec- ognized. Even more, albumin’s ability to protect RBC against induced hemolysis, stabilizing RBC against environmental insults including mechanical stress, had been reported previ- ously.36–38 Dramatic changes in RBC MF in the presence of albumin, shown in this work, illustrate the value of assess- ment of RBC mechanical properties in a native environment. For example, efficacy and survival of pRBC posttransfusion may be dependent not only on the unit’s RBC properties and storage media but also on the biochemical characteristics of a patient’s blood as well. Albumin, and possibly other plasma components, can alter RBC response to perturbations, as illustrated through changes in MF due to temperature- induced degradation and those due to membrane modifica- tions through MCD and DA. Potential for such modified responses should be accounted for in evaluation of hemolytic and sub-hemolytic damage to stored RBC, as this can be important both for validating any given MF test or protocol, as well as broadening the uses of MF testing to include investigations of RBC storage solutions and any role of the recipient’s physiology.
In conclusion, RBC MF profiles allow for a more compre- hensive assessment of the mechanical properties of RBC
membranes than do single-point measurement methods, with some indication that different MF parameters derived from a given MF profile can potentially offer different kinds of clin- ical relevance. Tracking MF, alone or in conjunction with other physiological properties and/or RBC ST, can provide additional information for physicians to factor into their judgments regarding transfusion and blood product use. Although the clinical significance of RBC MF remains to be established by further studies, the described method of assessing RBC MF profiles provides a particularly sensitive approach to evaluation of the cells’ membrane integrity and stability that prospective users are continuing to explore for both research and clinical applications.
ACKNOWLEDGMENTS
The authors thank the personnel of the University of Michigan Blood Bank, and in particular Theresa Downs, Supervisor, for her help in obtaining blood samples, and Robertson Davenport, Director, for his support and helpful discussions. The authors also thank Jed Gorlin and Kim Doeden of Memorial Blood Centers, collaborators on related work, for their support and assistance. The authors are employees of Blaze Medical Devices, a company that develops tests for RBC mechanical fragility, and which has funded all of the research reported in this article.
REFERENCES
- Bowersox JC, Hess JR: Trauma and military applications of blood sub- stitutes. Artif Cells Blood Substit Immobil Biotechnol 1994; 22: 145–57.
- Hess JR, Holcomb JB: Transfusion practice in military trauma. Transfus Med 2008; 18: 143–50.
- Spinella PC, Perkins JG, Grathwohl KW, et al: Risks associated with fresh whole blood and red blood cell transfusions in a combat support hospital. Crit Care Med 2007; 35: 2576–81.
- Doctor A, Spinella P: Effect of processing and storage on red blood cell function in vivo. Semin Perinatol 2012; 36: 248–59.
- Triulzi DJ, Yazer MH: Clinical studies of the effect of blood storage on patient outcomes. Transfus Apher Sci 2010; 43: 95–106.
- Spinella PC, Doctor A, Blumberg N, Holcomb JB: Does the storage duration of blood products affect outcomes in critically ill patients? Transfusion 2011; 51: 1644–50.
- van de Watering L: Red cell storage and prognosis. Vox Sang 2011; 100: 36–45.
- Napolitano LM, Corwin HL: Efficacy of red blood cell transfusion in the critically ill. Crit Care Clin 2004; 20: 255–68.
- Leal-Noval SR, Munoz-Gomez M, Arellano-Orden V, et al: Impact of age of transfused blood on cerebral oxygenation in male patients with severe traumatic brain injury. Crit Care Med 2008; 36: 1290–6.
- Lelubre C, Piagnerelli M, Vincent JL: Association between duration of storage of transfused red blood cells and morbidity and mortality in adult patients: myth or reality? Transfusion 2009; 49: 1384–94.
- Spinella PC, Carroll CL, Staff I, et al: Duration of red blood cell storage is associated with increased incidence of deep vein thrombosis and in hospital mortality in patients with traumatic injuries. Crit Care 2009; 13: R151.
- Aucar JA, Isaak E, Anthony D: The effect of red blood cell age on coagulation. Am J Surg 2009; 198: 900–4.
- Lacroix J, He´bert P, Fergusson D, et al: The Age of Blood Evaluation (ABLE) randomized controlled trial: study design. Transfus Med Rev 2011; 25: 197–205.
- Steiner ME, Assmann SF, Levy JH, et al: Addressing the question of the effect of RBC storage on clinical outcomes: the Red Cell Storage Duration Study (RECESS) (Section 7). Transfus Apher Sci 2010; 43: 107–116
MILITARY MEDICINE, Vol. 180, March Supplement 2015
Downloaded from publications.amsus.org: AMSUS - Association of Military Surgeons of the U.S. IP: 141.214.017.252 on Mar 10, 2015. Copyright (c) Association of Military Surgeons of the U.S. All rights reserved.
- Fergusson D, Hutton B, Hogan DL, et al: The age of red blood cells in premature infants (ARIPI) randomized controlled trial: Study design. Transfus Med Rev 2009; 23: 55–61.
- Fergusson D, Herbert P, Hogan DL, et al: Effect of fresh red blood cell transfusions on clinical outcomes in premature, very low-birth-weight infants: the ARIPI randomized trial. J Am Med Assn 2012; 308: 1443–51.
- Dern RJ, Gwinn RP, Wiorkowski JJ: Studies on the preservation of human blood. I. Variability in erythrocyte storage characteristics among healthy donors. J Lab Clin Med 1966; 67: 955–65.
- Pieracci FM, Moore EE, Chin T, et al: The age of transfused blood predicts hematocrit response among critically ill surgical patients. Am J Surg 2012; 204: 269–73.
- Dumont LJ, Zimring JC, Roback JD: Correlation of RBC Metabolomic Changes During Storage with RBC Survival After Transfusion in Human Autologous Donor/Recipients, AABB Conference 2012. Boston, MA. Transfusion 2012; 52: 12A.
- Cohen RM, Franco RS, Khera PK, et al: Red cell life span heterogeneity in hematologically normal people is sufficient to alter HbA1c. Blood 2008; 112: 4284–91.
- Dern RJ, Brewer GJ, Wiorkowski JJ: Studies on the preservation of human blood. II. The relationship of erythrocyte adenosine triphosphate levels and other in vitro measures to red cell storageability. J Lab Clin Med 1967; 69: 968–78.
- Zimmermann R, Heidenreich D, Weisbach V, Zingsem J, Neidhardt B, Eckstein R: In vitro quality control of red blood cell concentrates out- dated in clinical practice. Transfus Clin Biol 2003; 10: 275–83.
- Alfano K, Tarasev M: Investigating direct non-age metrics of stored blood quality loss. Internet J Med Technol 2011; 5: 1.
- Raval JS, Waters JH, Seltsam A, et al: The use of the mechanical fragility test in evaluating sublethal RBC injury during storage. Vox Sang 2010; 99: 325–31.
- Barshtein G, Manny N, Yedgar S: Circulatory risk in the transfusion of red blood cells with impaired flow properties induced by storage. Transfus Med Rev 2011; 25: 24–35.
- Burns JM, Yang X, Forouzan O, Sosa JM, Shevkoplyas SS: Artificial microvascular network: a new tool for measuring rheologic properties of stored red blood cells. Transfusion 2012; 52: 1010–23.
- Tarasev M, Alfano K, Chakraborty S, Bertholf M, Zubair A: Mechanical fragility as a potential time-independent measure of membrane integrity among stored RBC units. J Blood Disorders Transfus 2013; 4: 2.
- Tarasev M, Alfano K, Chakraborty S, Light L, Doeden K, Gorlin J: Similar donors—similar blood? Transfusion 2014; 54(3 Pt 2): 933–41.29. Sowemimo-Coker SO: Red blood cell hemolysis during processing. Transfus Med Rev 2002; 16: 46–60.
- Sowemimo-Coker SO: Red blood cell hemolysis during processing. Transfus Med Rev 2002; 16: 46–60.
- Rodrigues R, Faustino V, Pinto E, Pinho D, Lima R: Red Blood Cells deformability index assessment in a hyperbolic microchannel: the diamide and glutaraldehyde effect. In WebmedCentral BIOMEDICAL ENGINEERING, 4(8), WMC004375. Available at http://www.web medcentral.com/wmcpdf/Article_with_review_WMC004375.pdf; Accessed July 15, 2015.
- Stadnick H, Onell R, Acker JP, Holovati JL: Eadie-Hofstee analysis of red blood cell deformability. Clin Hemorheol Microcirc 2011; 47: 229–39.
- Tarssanen L: Hemolysis by ultrasound. A comparative study of the osmotic and ultrasonic fragility tests. Scand J Haematol Suppl 1976; 29: 1–59.
- Ramirez-Arcos S, Mastronardi C, Perkins H, et al: Evaluating the 4-hour and 30-minute rules: effects of room temperature exposure on red blood cell quality and bacterial growth. Transfusion 2013; 53: 851–9.
- Eastlund T, Van Duren A, Clay ME: Effect of heat on stored red cells during non-flow conditions in a blood-warming device. Vox Sang 1999; 76: 216–9.
- Gershfeld NL, Murayama M: Thermal instability of red blood cell mem- brane bilayers: temperature dependence of hemolysis. J Membr Biol 1988; 101: 67–72.
- Zavodnik YB, Piletskaia TP, Stepuro II: [Mechanical lysis of human erythrocytes. Membrane stabilization by plasma proteins]. Ukr Biokhim Zh 1991; 63: 72–8.
- Rudenko SV, Nipot EE: Protection by chlorpromazine, albumin and bivalent cations against haemolysis induced by melittin, [Ala-14] melittin and whole bee venom. Biochem 1996; 317(3): 747–54.
- Kameneva MV, Antaki JF, Yeleswarapu KK, Watach MJ, Griffith BP, Borovetz HS: Plasma protective effect on red blood cells exposed to mechanical stress. Asaio J 1997; 43: M571–5
MILITARY MEDICINE, Vol. 180, March Supplement 2015
Downloaded from publications.amsus.org: AMSUS - Association of Military Surgeons of the U.S. IP: 141.214.017.252 on Mar 10, 2015. Copyright (c) Association of Military Surgeons of the U.S. All rights reserved.